Introduction
Energy conservation has become a global trend and a concern for industries worldwide. As energy costs continue to rise and environmental regulations become more stringent, process engineers play a crucial role in designing energy-efficient plants that minimize energy consumption and reduce operating costs.
This can be shown in different forms when carrying out the process design. In this article, we shall go through some techniques commonly followed by a process engineer to deliver energy-efficient plant, unit, and equipment and the major concerns or caveats we should consider when applying these techniques.
If you’d like to see the content of this article as a video, you can check out our YouTube video. Otherwise, you can continue reading this article:
Importance of energy conservation
Energy conservation is a real challenge that faces any plant owner. This is because an inefficient plant would mean much more power and utility consumption, leading to an increase in the plant operating cost. In addition, failing to apply proper energy conservation when carrying out the process design would typically mean a larger capacity in utility systems.
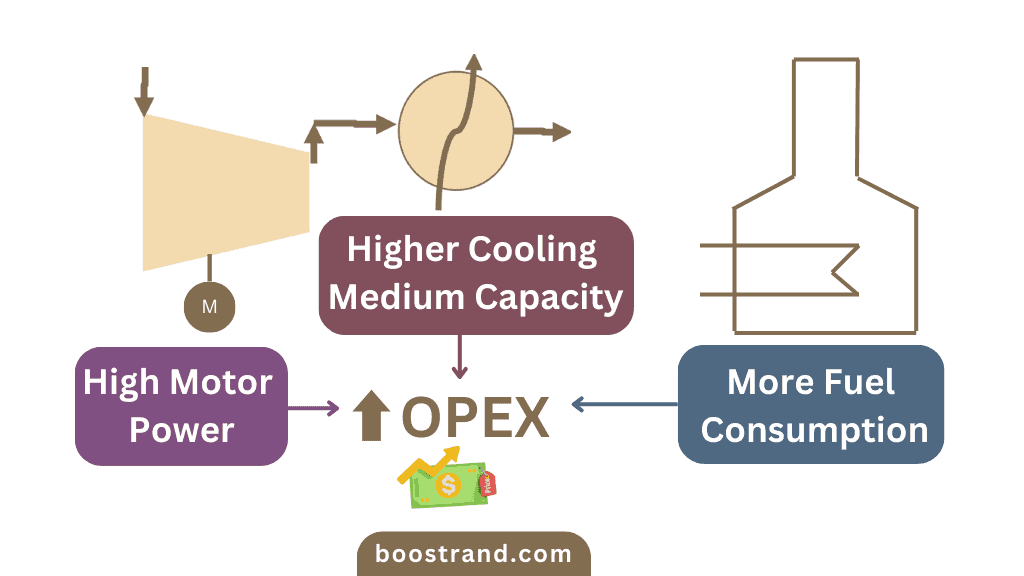
For example, if we fail to apply heat integration between hot and cold process streams, this would mean that we need more cooling water to cool down the hot stream and more steam or hot oil to heat up the cold streams. Increasing the cooling water requirements would mean that we shall need a larger cooling tower and cooling water pump for the cooling water system. As for heating requirements, we shall need a larger boiler and water treatment system for the steam system. If we are using hot oil for heating, we shall need a hot oil heater with a larger duty for the hot oil system. All this means more fuel consumption, which is a large operating cost.
Role of a process engineer in energy conservation
That’s why a process engineer must optimize the energy conservation in any plant, as this shall significantly reduce the plant operating cost, and yield utility systems with lower capacities, which is also considered as a reduction in the fixed cost.
So how can a process engineer apply energy conservation practices in process design? Let’s see.
Forms of energy conservation
Proper Process Design
A proper process design is the shortest and most straightforward way to consider energy conservation. When a process engineer puts operating cost in mind, he could easily save a lot of effort in energy conservation.
Example 1: Optimizing Tower Reflux and Vapor Boilup Ratios
This is probably the most famous and most common type of energy conservation in any process. As we highlighted in the article talking about tower design parameters, the tower’s reflux ratio and vapor boilup can highly enhance the mass transfer on the tower trays. This can significantly reduce the number of stages in a tower, which would help reduce the tower’s height, which shall significantly reduce the total tower fixed cost.
However, a higher reflux ratio would lead to a larger condenser duty, which means a larger exchanger and more cooling medium capacity. This means more cooling water pump or cooling tower capacity or more propane refrigeration capacity or higher air cooler power to be consumed. If we are talking about reboiler section, things are even expected to get worse, as we are dealing here with heating media such as steam or hot oil. These are commonly generated by fired heaters or boilers which are expensive systems that need to burn a lot of fuel.
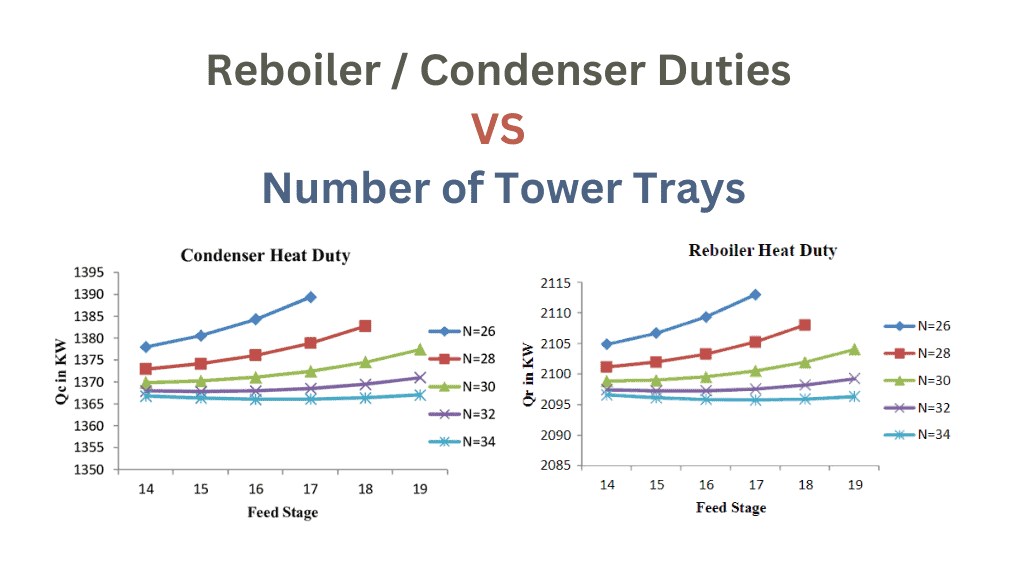
In the above example, we see that at a larger number of stages (N > 30), we needed a very small increase in reboiler and condenser duties to reduce the number of stages from 34 to 30, so here it would be highly feasible to pay for this slight increase in operating cost against the reduction of fixed cost. However, we needed more than double the increase in duties to reduce the number of stages from 30 to 26, which means much higher operating cost. If we are targeting a number of trays of 22, we may end up with huge reboiler and condenser duties that won’t deem this reduction as feasible compared to the huge increase in operating costs. You can check out the source of whole study in this link.
That’s why a process engineer should ensure proper optimization between different process parameters. In the beginning, raising the reflux and boil-up ratios can yield in significant reduction in the tower’s fixed cost. However, after certain values, increasing the reflux ratio won’t highly affect the separation degree in a tower while the impact would be high on tower’s overhead and bottom systems. That’s why optimization should be considered here.
Example 2 Absorber Pressure
For example, any absorption process favors a high pressure. This would increase the recovery of the impurity to be removed from the main gas. So if we increased the pressure, this would favor the operation of the absorber.
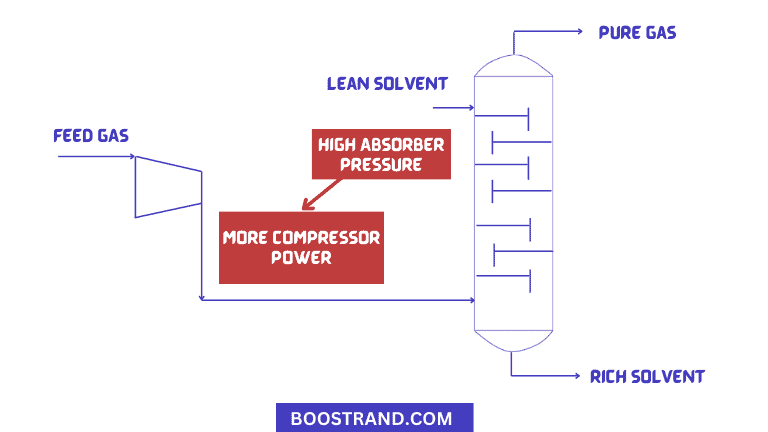
However, excessively increasing the pressure would lead to a larger compressor power required to deliver the gas to the absorber, which is commonly a large operating cost. So here we should only consider the operating pressure that would deliver enough separation and yield on-spec product so that the absorption process achieves its goal. So optimizing the absorber’s pressure is important in order to optimize both the feed gas compressor and lean solvent pump.
Example 3: Pump Discharge Pressure Drop
This is even more clear when considering a reasonable pressure drop in the piping and equipment on pump discharge. An undersized pipe or an exchanger would lead to a high pressure drop. This high pressure drop is just a power loss. The pump needs to compensate for this power. If the pressure drop is very excessive, the pump may work in the minimum flow region which is a low efficiency region. All these are power losses. If you like to know more about pump hydraulic calculations, you can check out our pump hydraulics course.
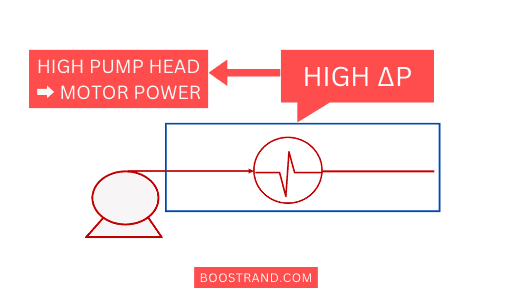
So here a process engineer needs to carry out hydraulic calculations in an optimized way. And that’s why most companies consider pressure drop criteria in pump and compressor discharge to keep the pressure drop within acceptable limits without considering a very large pipe size.
Example 4: Depending on Gravity as a Driving Force
As a process engineer is expected to optimize the pump hydraulic calculations and the pressure to be achieved, he can even sometimes eliminate the need of a pump. This is commonly applied in tower reboiler systems where the driving force can be adjusted by tower and reboiler elevations as well as reducing the pressure drop in the reboiler and the piping to a minimum. Although this can increase the fixed cost of column foundations and require a heavier steel structure, the elimination of the reboiler pump would be worth it in most cases.
Energy integration
Integrating hot and cold streams is one of the most famous practices a process engineer thinks about when designing a process. Most processes would have hot streams and cold streams. We can integrate between both streams in order to reduce the heating and cooling duties.
For example, if we are designing a process where the feed to a reactor or to a tower is required to be heated, and in most cases the product is required to be cooled, we can heat the feed with the effluent or the product. This can significantly reduce the heating duty on the feed heater and the cooling duty in the product cooler.
Example: Atmospheric Distillation Pre-heat Train
A famous example is the atmospheric distillation unit where we are dealing with crude oil that needs to be heated from ambient temperature to more than 350 C. If we depend only on a fired heater to heat up the crude, this would result in a huge heater duty, which would require enormous fuel consumption and operating cost, in addition to the huge fixed cost of the fired heater. On the other hand, in order to cool down the tower’s products before being sent to storage, we would need a huge cooling system to cool them down from 100-350 C to ambient temperature. This would also mean a huge loss of energy in addition to providing enormous utility systems.
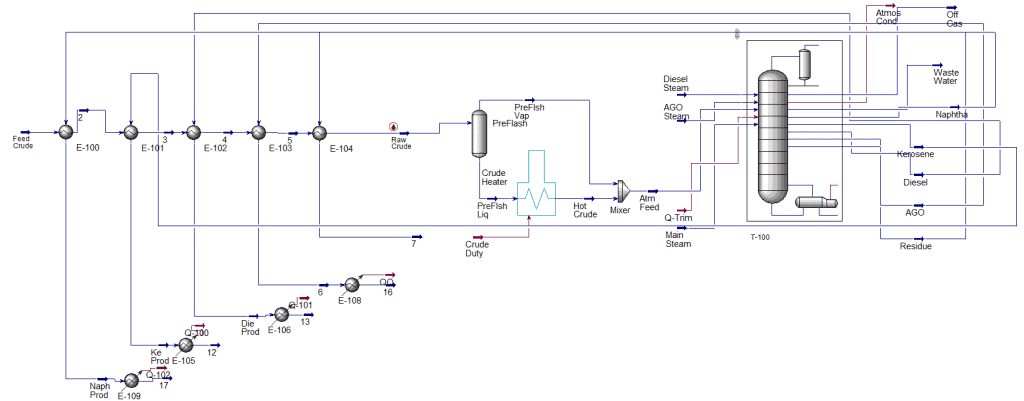
Here, we are dealing with a huge number of streams, there are several products and pump-around streams at different flow rates and temperatures, we want to maximize energy recovery by utilizing all these streams in order to raise the temperature of the feed crude as much as possible.
This is commonly carried out in the preheat train where a network of heat exchangers is placed in order to recover the heat from products and increase the temperature of the feed crude. In order to optimize the placement of heat exchangers at preheat train, a pinch analysis is carried out.
This analysis requires a deep understanding of heat transfer, with respect to heat duties and temperature approaches. Using simulation tools like HexTran in addition to validating the design using HTRI is necessary to get the required temperatures and specifying the duty of each exchanger that would lead to efficient energy integration along with reasonable exchanger sizes.
This preheat train can raise the temperature of the crude to 230-270 C, which represents the majority of the required heating duty. This means that we shall need the fired heater just to raise the temperature from 230 C to 350 C.
Process Engineering Masterclass
Become a Professional Process Engineer, discover process engineering career, role, activities and common practices with access to most of the courses here.
So here this is a great example of energy conservation, by using a preheat train, we have significantly reduced the fuel consumption to raise the feed crude temperature, we have a significantly much smaller heater, and we cooled down the products as well, which shall significantly reduce the capacity of the cooling system required to cool down these products.
Generating power
Energy recovery is not just related to heat transfer, but it is also commonly used in mechanical power. A famous example is the use of turboexpanders to generate mechanical power that can drive gas compressors.
Turboexpanders are mechanical devices that remove energy from a high-pressure process gas stream, resulting in a simultaneous drop in both pressure and temperature. The removed energy is converted into mechanical energy. Generator-loaded turboexpanders convert the recovered energy into electricity, which can be used within the plant or sold back to the local electrical grid. Otherwise, if we have compressors in our process, they can drive the compressors.
So here, instead of using electric motor and consuming megawatts of electricity, we can use the energy from turboexpanders. This shall result in significant energy savings and much less gas to be burnt for power generation, which means less carbon emissions.
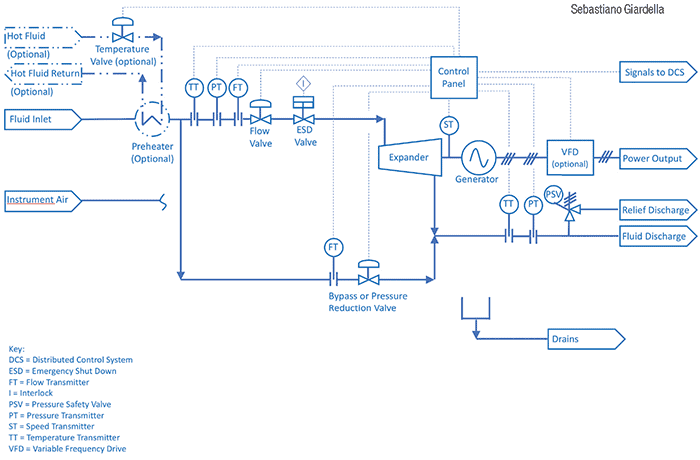
Turboexpanders are commonly used in various energy conservation applications in the process industry such as cryogenic pressure let down, dew point control, C3/C4 recovery, C2 recovery, and ethylene processing.
Choosing Efficient Equipment
Another form of energy conservation is to choose the pieces of equipment that shall ensure the lowest power consumption. For example, when we talk about rotating equipment, this is commonly reflected in their efficiency. A compressor with a low efficiency would lead to more power consumption, which would mean more operating cost. A fired heater with a low efficiency would mean that it would need more fuel to achieve the required duty.
So here, when selecting the equipment, it is not just a matter of choosing the minimum fixed cost. This should be optimized with the expected operating cost which shall continue on the long run.
Process Engineering Masterclass
Become a Professional Process Engineer, discover process engineering career, role, activities and common practices with access to most of the courses here.
Control Methods
In addition to the above techniques, process control can significantly save a lot of power. A famous example is the use of variable speed control in pumps and compressors.
Variable speed drives (VSD) allow the speed of pumps and compressors to be adjusted to match the process demand. This is much more energy efficient than running them at constant speed and throttling the flow with control valves. When we need much less flow from the pump of the compressor, we can reduce the speed of the impeller instead of throttling the discharge control valve. This shall dramatically reduce the power consumed by the pump or compressor as power is proportional to the cube of speed.
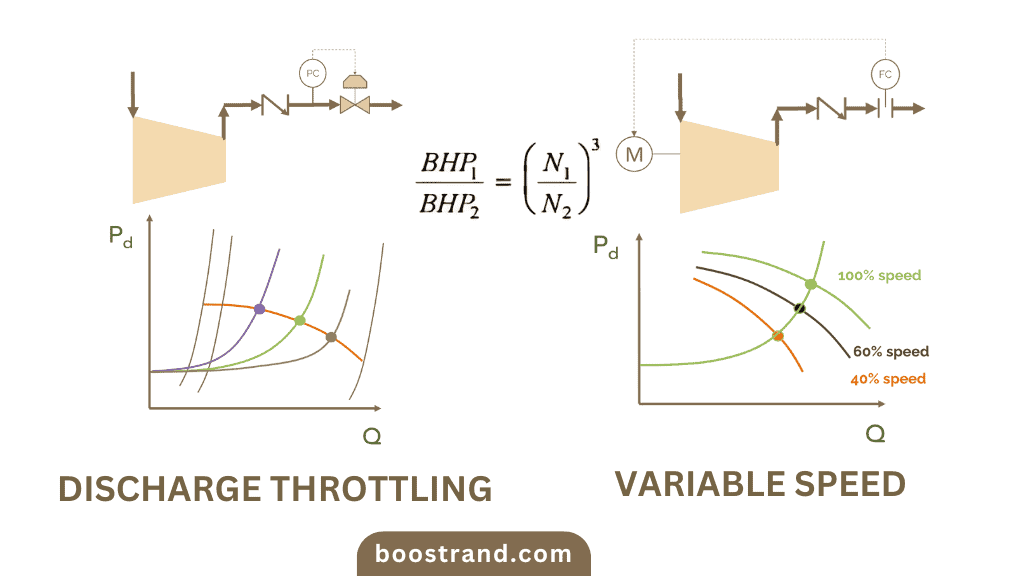
For example, reducing the speed by 20% can result in nearly 50% energy savings. However, if we applied discharge throttling, a centrifugal pump will go to a less flow region, give higher head at a lower efficiency, which will not lead to any power savings.
Although they have much higher fixed cost and maintenance requirements, using motors with variable speed drive can save a lot of energy, which means much less operating cost on the long run if the pump or compressor are expected to consume high power.
Drawbacks of Conservation methods
So we have seen several examples showing how we can maximize energy savings when carrying out our process design. However, applying these techniques usually have drawbacks. Let’s talk more about that.
Higher Fixed Cost
Usually reducing the operating cost shall have a negative impact on fixed cost. For example, using variable speed drive motors is much more expensive than fixed speed drive motors. Turboexpanders have a very high initial cost and required complicated equipment procedures as well. Fired heaters or rotating equipment will be more expensive if they are highly efficient. This is a common drawback when using energy saving equipment. However, this high fixed cost can be returned as savings in operating cost if the proper equipment was chosen.
This is not just related directly to equipment cost. This can also affect the whole system serving the equipment. For example, in a centrifugal multistage compressor, we can add more compressor stages with intercooling. This shall highly increase the overall compressor’s efficiency as it’s highly affected by the inlet temperature to the compressor. That’s why it’s common to add more than one stage if the outlet temperature of a compressor increases to around 150 C. However, adding more compressor stages would mean more compressor equipment, more intercoolers, more discharge scrubbers, more foundations, and so on.
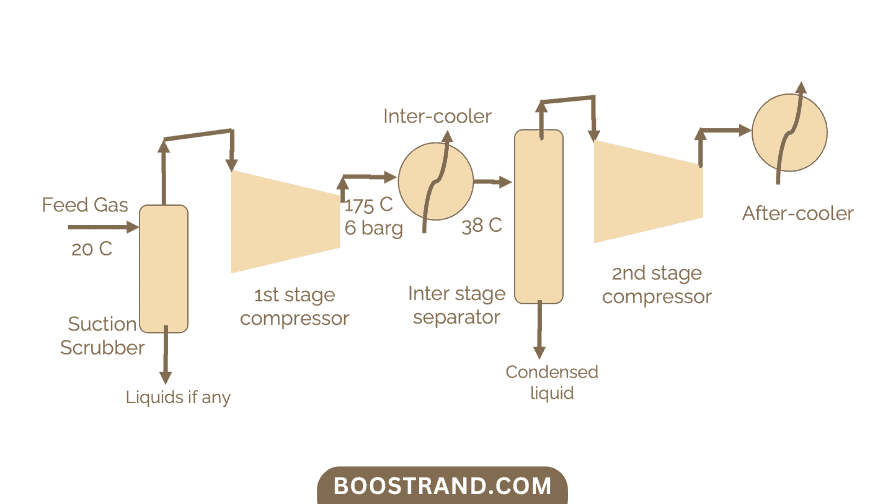
We can also increase the efficiency of intercooling by using water coolers that would even reduce the outlet temperature of each stage to a temperature less than that achieved by the air cooler. This would significantly increase the efficiency of the next inlet compressor stage. However, if there is no enough cooling water available in the plant to achieve this intercooling, we would need to include a new cooling water system, which won’t be feasible in most cases.
Engineering difficulties
The other drawback is related to the difficulties that the engineering team may face when carrying out the process design.
Heat Recovery VS Temperature Approach
For example, when designing a preheat train, issues with temperature approach and oversizing of an exchanger are very common. This is because the lower the temperature difference between the hot and cold sides of an exchanger, the higher the heat transfer area required, which means a larger exchanger size. This is explained detail in our article talking about the heat exchanger design parameters.
Suppose that we have a hot stream is 180°C and we want to cool it to 150 C using a cold stream at inlet temperature of 170°C (stream A), this means a low driving force, which can lead to a significantly a heat exchanger with a high heat transfer area due to the low MTD. On the other hand, if we used another stream B at 120 C, this would lead to much more efficient heat transfer, which means a much smaller heat exchanger. If we didn’t apply energy integration and just used a water cooler, we can end up with a tiny heat exchanger.
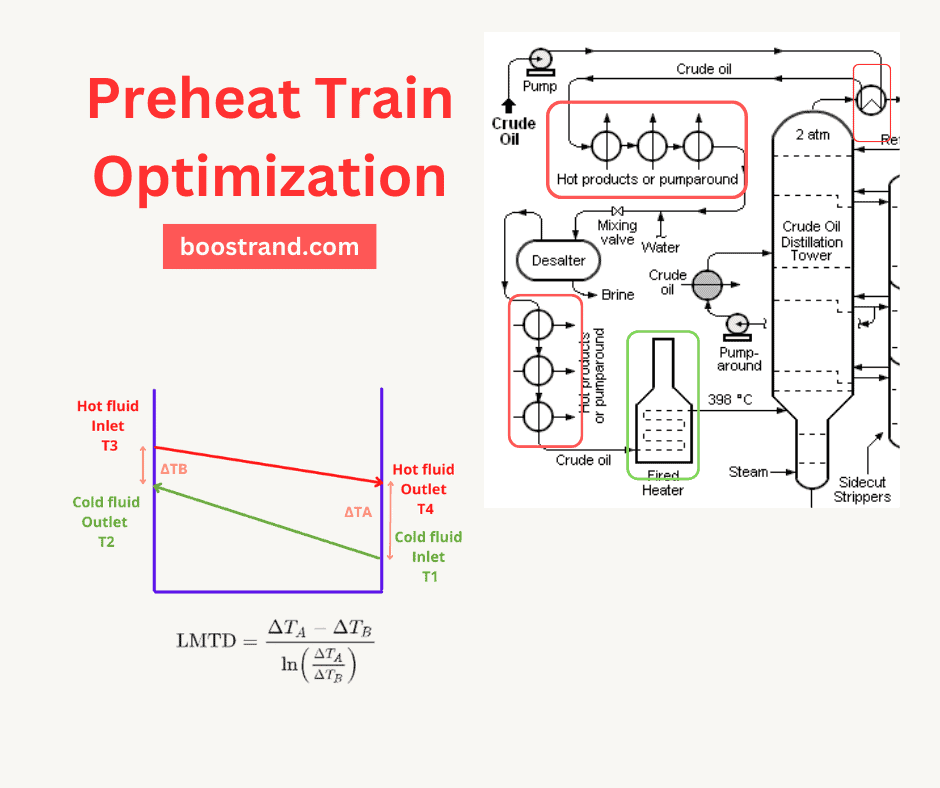
So here, choosing the proper process scheme that ensures maximum heat recovery while providing a reasonable exchanger design can be challenging in many cases.
Adjusting Hydraulics VS Elevation requirements
Another example of engineering difficulties engineers may face is when using once-through reboilers in tower configuration. As highlighted above, this shall eliminate the need for a pump, which is great. However, this shall also lead to a larger tower elevation to ensure proper driving force. This means more foundations and complex piping design for this portion and in some cases, it may be difficult to achieve the required design that provides the proper recirculation from the tower to the reboiler to the tower again.
So as we are putting energy savings in mind, we should assess the difficulties we shall face and ensure they are well addressed.
Conclusion
Process engineers play a vital role in ensuring that plants are designed to be energy-efficient, thereby reducing operating costs and minimizing environmental impacts. By employing techniques such as Pinch Technology for heat integration, optimizing process parameters, using variable speed drives, and carefully selecting equipment, significant energy savings can be achieved and the plant operating cost can be reduced. This can lead to a greater profit on the long run.
However, a process engineer should also consider the expected caveats when using these techniques such as increased fixed cost, complicated maintenance procedure or operational issues in order to deliver a proper process design.
Start your Career
Access Process Engineering Introduction Course
Share this:
[…] creation of a PFD is typically based on the output of process simulation software. We have discussed plant simulation’s role in a project in the previous article. In a nutshell, process simulation involves using specialized software to model and analyze the […]